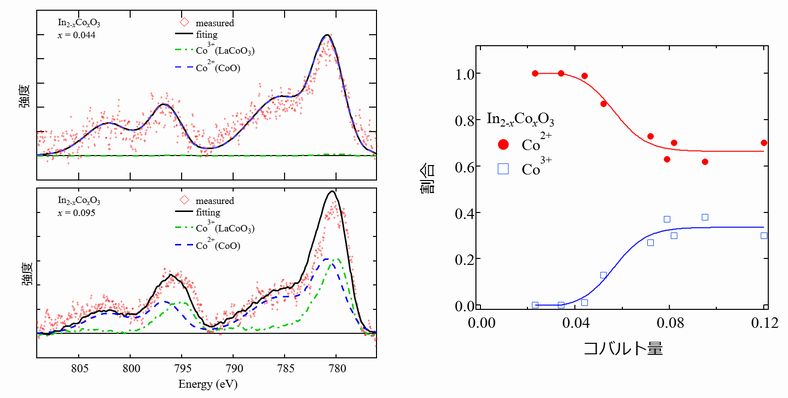
In In2-xCoxO3, where some In in In2O3 is substituted with Co, we investigated the Co ions’ 24d and 8b site occupancy, valence states, and spin states. Through Rietveld analysis of the product’s X-ray powder diffraction patterns, it became clear that Co2+ and Co3+ ions tend to occupy 24d sites and 8b sites respectively. X-ray photoelectron spectroscopy analysis shows that Co2+ ions are dominant in the low-doping region (x<0.044), while Co3+ ions appear in the high-doping region (x>0.052). Through magnetic susceptibility measurements, the effective Bohr magneton number (p_eff) in the low-doping region is approximately 3.8, which agrees with the value for high-spin (S=3/2) state Co2+. The p_eff decreases in the high-doping region where Co3+ ions appear. These results indicate that Co3+ ions are in either low-spin (S=0) or intermediate-spin (S=1) states.
[Note: The image shows spectroscopic data and graphs illustrating the concentration dependence of Co2+ and Co3+ ions, along with their corresponding energy spectra.]
H.Kumagai, Y.Hara, K.Sato, Journal of Magnetism and Magnetic Materials Volume 489, 1 November 2019, 165358, “Site occupancy, valence state, and spin state of Co ions in Co-doped In2O3 diluted magnetic semiconductor”, https://doi.org/10.1016/j.jmmm.2019.165358
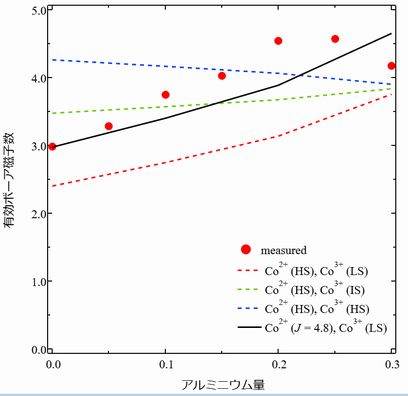
We investigated the valence and spin states of cobalt ions, as well as room temperature magnetization in In1.9-xAlxCo0.1O3. X-ray photoelectron spectroscopy revealed that samples without Al contained a mixture of Co3+ and Co2+. As the Al content increased, the proportion of Co2+ increased, eventually resulting in Co2+ only. Magnetic susceptibility analysis showed that for Co2+, both spin and orbital states were magnetized, while Co3+ was in a low-spin state. Through vacuum annealing, Co3+ converted to Co2+, and we found that the higher the conversion ratio from Co3+ to Co2+, the stronger the magnetization became at room temperature.
H.Kumagai, Y.Hara, K.Sato, Journal of Magnetism and Magnetic MaterialsVolume 564, Part 2, 15 December 2022, 170150, “Chemical-substitution dependence of magnetization strength in room- temperature ferromagnetism of dilute magnetic oxides: Valence and spin state of slightly doped cobalt in (In,Al)2O3”, https://doi.org/10.1016/j.jmmm.2022.170150
- Study of (La,Sr)CoO3 Showing Magnetic Shape Memory Effect
- Study of Cobalt Spin States in Perovskite Oxides
- Past Research Achievements: Study of Electro-Optical Effects in Dielectric Materials
- Past Research Achievements: Study of Magnetostriction and Magnetization in LaCoO3
- Relationship Between Past and Current Research
Study of (La,Sr)CoO3 Showing Magnetic Shape Memory Effect
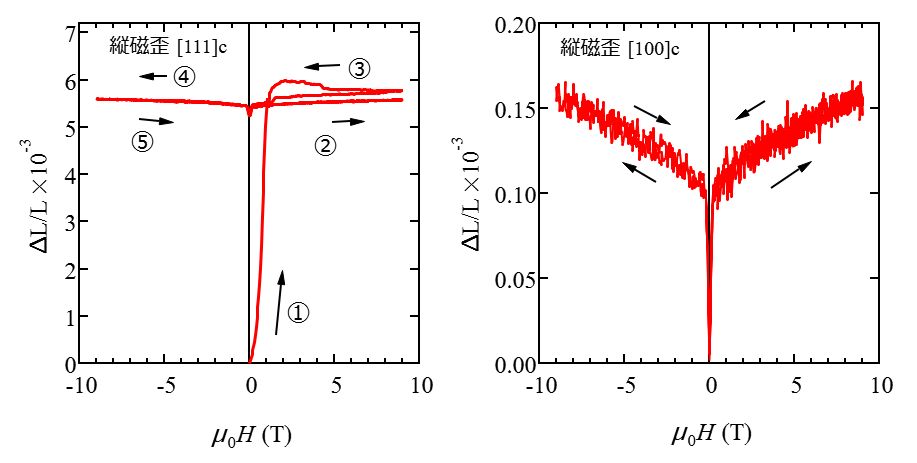
We investigated the crystal orientation dependence of magnetostriction in the double perovskite La0.8Sr0.2CoO3, which exhibits a magnetic shape memory effect. When a magnetic field was applied along the [111]c and [110]c directions of the distorted cubic crystal, the magnetostriction showed significant hysteresis and residual strain. On the other hand, when the magnetic field was applied along the [001]c direction, the magnetostriction showed neither hysteresis nor residual strain. The large hysteresis and residual strain in the [111]c and [110]c directions could be explained by the rearrangement of crystal domains with the [111]r direction of the rhombohedral crystal as the magnetic hard axis. The absence of hysteresis and residual strain in the [001]c direction magnetostriction is thought to be due to the equal projection of all domain [111]r axes onto the [001]c direction, making domain rearrangement impossible. Thus, the crystal orientation dependence of magnetostriction in La0.8Sr0.2CoO3 can be explained by crystal domain rearrangement.
[Note: The image shows two graphs displaying magnetostriction data along [111]c and [100]c directions, with arrows indicating measurement sequences and showing different hysteresis behaviors.]
K. Sato, K. Minagawa, M. Nakamura, T. Ichikawa, Y. Hara, K. Nakaoka, Y. Kobayashi, K. Asai, Journal of Alloys and Compounds 752 327-331,2018, “Crystal orientation dependence of magnetostriction of twinned perovskite cobalt oxide” https://doi.org/10.1016/j.jallcom.2018.04.139
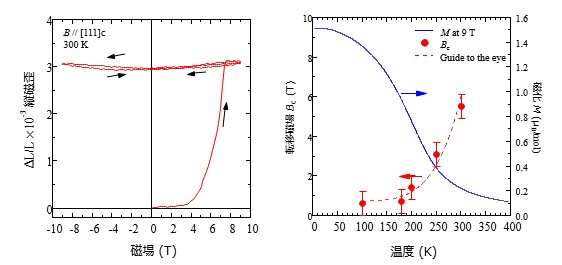
For the same material mentioned above, we reported that it shows magnetic shape memory effect even at room temperature. Although the critical magnetic field is relatively large at 6 T, this is the first report of such an effect in oxide materials.
A. Yokosuka, H. Kumagai, M. Fukuda, K. Ando, Y. Hara, and K. Sato, JAIP Advances 10, 095217 (2020);”Room temperature magnetic shape-memory effect in strontium-doped lanthanum cobaltite single crystals”, https://doi.org/10.1063/5.0021751
Study of Cobalt Spin States in Perovskite Oxides
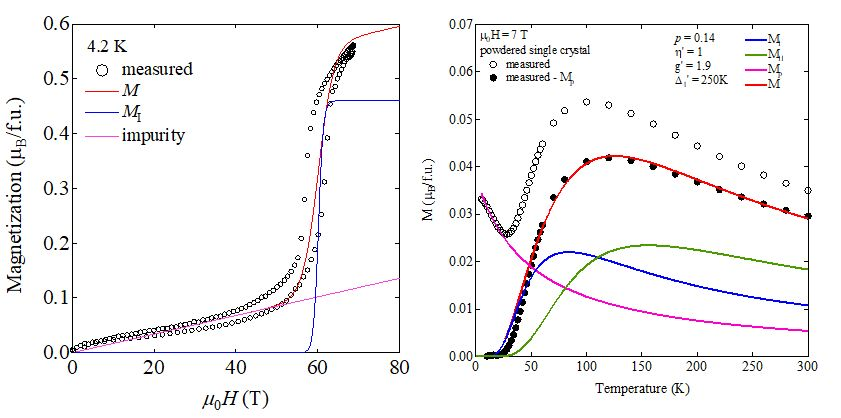
We investigated the spin states of Co ions in the perovskite oxide LaCoO3 system through magnetization measurements up to a maximum magnetic field of 67 T. LaCoO3 showed a magnetic transition near 60 T at 4.2 K. This is thought to be due to some Co ions transitioning from a low-spin to a high-spin state. The remaining cobalt ions do not transition up to 67 T, but they are believed to contribute to the anomaly in magnetization at 100 K through a transition from low-spin to intermediate-spin states. In (La,Sr)CoO3, where some La is substituted with Sr, it is thought that an intermediate spin state of Co3+ centered around Co4+ is formed. Additionally, in La(Co,Rh)O3, where some Co is substituted with Rh, we found that the magnetic transition observed in LaCoO3 shifts to the low magnetic field side, and the high-spin state becomes stable.
Keisuke Sato, Akira Matsuo, Koichi Kindo, Yoshihiko Kobayashi, and Kichizo Asai, J. Phys. Soc. Jpn. 78, 093702 (2009); “Field Induced Spin-State Transition in LaCoO3” https://doi.org/10.1143/JPSJ.78.093702
Keisuke Sato, Akira Matsuo, Koichi Kindo, Yoshihiko Kobayashi, and Kichizo Asai, J. Phys. Soc. Jpn. 80, 104702 (2011); “Spin State of Co Ions in Lightly Doped Strontium LaCoO3: Via Study of High-Field Induced Spin State Transition” https://doi.org/10.1143/JPSJ.80.104702
Keisuke Sato, Akira Matsuo, Koichi Kindo, Yoshiaki Hara, Kanichiro Nakaoka, Yoshihiko Kobayashi, and Kichizo Asai, J. Phys. Soc. Jpn. 83, 114712 (2014); “Field-Induced Spin-State Transition in LaCo1−xMxO3 (M = Al, Ga, Rh, and Ir)” https://doi.org/10.1143/JPSJ.80.104702
Past Research Achievements: Study of Electro-Optical Effects in Dielectric Materials
In addition to current magnetic materials research, while I was at Fujitsu Laboratories, I conducted research on electro-optical effects in dielectric materials, particularly in lanthanum-doped lead zirconate titanate (PLZT). At Fujitsu Laboratories, we fabricated PLZT epitaxial thin films with different crystal orientations – (100), (101), and (111) – and clarified the crystal orientation dependence of their electro-optical effects. Through this research, we demonstrated that the electro-optical effect in PLZT can be explained by the rotation of refractive index ellipsoids accompanying the switching of polar clusters. These research findings were published in the following peer-reviewed papers:
- K. Sato et al., J. Appl. Phys. 102, 054104 (2007). https://doi.org/10.1063/1.2774004
- K. Sato et al., Appl. Phys. Lett. 87, 251927 (2005). https://doi.org/10.1063/1.2147722
While our current laboratory focuses on magnetic materials research, my past research experience with dielectric materials provides a broad perspective for understanding and applying functional materials properties.
Past Research Achievements: Study of Magnetostriction and Magnetization in LaCoO3
LaCoO3 is known to be in a nonmagnetic low-spin state (S=0) at low temperatures, but exhibits a magnetic excited state as temperature increases. To clarify the details of this magnetic state, we measured the magnetostriction of LaCoO3 in pulsed magnetic fields up to 35 T. The magnetostriction was proportional to the square of the magnetic field and could be well explained by a spin-state transition model between the nonmagnetic ground state and magnetic excited state. Assuming g-factor and orbital reduction factor to be 3.35 and 1 respectively, the temperature dependence of magnetostriction could be well reproduced with an excitation energy of 140 K. While these parameters agree with previous ESR measurement results, they cannot explain the magnitude of magnetization. Comparing the pressure dependence of magnetostriction and magnetization revealed that elastic energy expressed only by lattice volume is insufficient to quantitatively describe the magnetoelastic phenomena in LaCoO3.
K. Sato et al., J. Phys. Soc. Jpn. 77 (2008) 024601. “High-Field Magnetostriction of the Spin-State Transition Compound LaCoO3” https://doi.org/10.1143/JPSJ.77.024601
Relationship Between Past and Current Research
In addition to current research on magnetic materials, I have previously worked on studies involving dielectric materials and cobalt spin states in the perovskite oxide LaCoO3.
In dielectric materials, we clarified the crystal orientation dependence of the electro-optical effect in lanthanum-doped lead zirconate titanate (PLZT). Through this research, we demonstrated that the electro-optical effect in PLZT can be explained by the rotation of refractive index ellipsoids accompanying the switching of polar clusters. This finding is now being applied to understanding the magnetic-field-induced strain anisotropy in (La,Sr)CoO3 and studying the effects of Co addition in transparent oxide In2O3.
From magnetostriction measurements of LaCoO3 under pulsed high magnetic fields, we investigated how Co3+ ion spin-state transitions affect magnetoelastic effects. This research suggested that elastic energy expressed only by lattice volume is insufficient for quantitatively describing magnetoelastic phenomena. This insight has led to developments in our current research on magnetic shape memory effects in (La,Sr)CoO3.
In this way, my experience in property evaluation of dielectric materials and perovskite oxide LaCoO3, along with perspectives focused on spin states and crystal orientations, is being applied to current magnetic materials research. I believe that research experience across diverse material groups will contribute to the development and functional exploration of new magnetic materials.